One of the world’s greatest environmental challenges can be summarised in three words: plastics are forever. The spread of plastic has been widely documented around the globe, and there have many efforts to quantify plastic production. In 2017, it was estimated that 8.3 billion metric tonnes of plastic had been manufactured since mass production began in the 1950s – and production is only accelerating.<super-script>1<super-script>
A less visible – and less understood – threat to the planet and its inhabitants comes in the form of small, microscopic (<5mm), synthetic polymers. These persistent particles can be generated by two main routes: from the degradation of bulk plastic litter, and the intentional addition to consumer products such as cosmetics, cleaning products. While researchers continue to gauge the impact of microparticles and nanoparticles (MPs and NPs) on the environment and human health, regulatory bodies contemplate their next moves. Restricting the intentional use of nanoplastics and microplastics can be considered a ‘low-hanging fruit’, and the European Chemicals Agency (ECHA) has already made moves towards this goal. While ECHA have acknowledged the significant knowledge gap related to the hazards of NPs, they have stated that the risks arising from intentional uses of MPs are not adequately controlled. Similarly, the World Health Organization has called for more research into MPs and NPs, voicing particular concerns for communities without adequate water and sewage treatments that remove MPs/NPs.<super-script>2<super-script> In 2019, ECHA submitted a restriction proposal, indicating their intention to prepare a restriction dossier related to the intentional use of MPs and NPs.<super-script>3<super-script>
The implementation of such regulations and relevant research would need to be supported by techniques for particle isolation, and size, concentration and chemical identity analysis. To provide an insight into the suitability of analytical techniques for NP and MP analyses, researchers from across six countries embarked on a collaborative study. Their work explored the landscape of techniques available for measuring size and mass-based concentration and was published in the Journal of Colloid and Interface Science in April 2021.<super-script>4<super-script>

Ten analytical techniques challenged with different mixtures of microplastics and nanoplastics
Caputo et al. (2021) challenged the capability of techniques to determine nanoparticle size distribution and mass-based concentration in a range of samples. The experiments were conducted using mixtures of NIST-traceable polystyrene standards, selected for their well-defined and reproducible properties. As the fourth most used synthetic polymer in consumer products (after polyethylene, polypropylene and polyethylene terephthalate), polystyrene is also a relevant material of interest. Samples contained standard particles of varying sizes and proportions, to challenge the techniques in different ways:
- Monomodal samples (100, 152, 203 and 240 nm). Tested techniques for their ability to measure size and mass-based concentration.
- Polydisperse samples (quadrimodal mixtures in the range of 100-250 nm). Theoretically, these samples were well within the range of applicability of each technique tested.
- Trimodal mixtures containing 60 nm particles (60, 100 and 150 nm particles). Aimed to assess lower limits of detection.
- Samples containing bimodal mixtures of 220 nm and 2 µm particles in varying ratios were assessed to determine techniques suited to detecting MPs in the presence of NPs.
Ten different sample mixtures were applied to the following techniques: dynamic light scattering (DLS), multi-angle dynamic light scattering (MADLS), transmission electron microscopy (TEM), nanotracking analysis (NTA), static light scattering (SLS), tunable resistive pulse sensing (TRPS), asymmetric flow field flow fractionation (AF4-MALS), centrifugal liquid sedimentation (CLS), flow cytometry (FCM) and nano flow cytometry (nFCM).
To be consistent with ECHA requirements, the following parameters were reported:
- Mass-based particle size distribution (PSD, ng/mL)
- Total mass concentration (ng/mL)
To enable direct comparisons, data for most techniques had to be converted from number-based to mass-based parameters.
Varying degrees of difficulty: Monomodal vs polydisperse samples
As expected, monomodal samples were easier to characterise than polydisperse samples; mode diameters of monomodal samples measured by most techniques (NTA, TRPS, MADLS, nFCM and AF4MALS) were in close agreement, while major differences in detection capabilities were observed for techniques faced with polydisperse samples. Despite their widespread use in nanoparticle analysis, DLS, MADLS and NTA were unable to clearly resolve polydisperse samples. This has been shown previously and has significant implications for nanoplastic analysis where heterogenous populations are to be expected.<super-script>5,6<super-script>
ECHA’s requirement for mass-based measurements introduces additional challenges; as seen for the quadrimodal samples, the conversion of number-based PSD to mass-based measurements compromised the resolution of most techniques to varying degrees.
Testing lower and upper limits of detection
Pushing the lower limits: Only TRPS and nFCM clearly identified all subpopulations in the sample containing 60 nm particles in line with the expected weight distributions.
Testing the upper limits: The final samples, containing 220 nm and 2 µm particles, were included to allow an assessment of the upper limit of applicability. Additionally, the authors hoped to determine which technique/s would be suited to characterising micro-sized particles 2 µm in the presence of nanoparticles. The ratio of 220 nm and 2 µm particles, respectively, were as follows: 50:50, 90:10, and 99:1 (%w/w). Most techniques were excluded from this part of the study for different reasons, leaving the following methods for analysis: DLS, TRPS, CLS, and SLS.
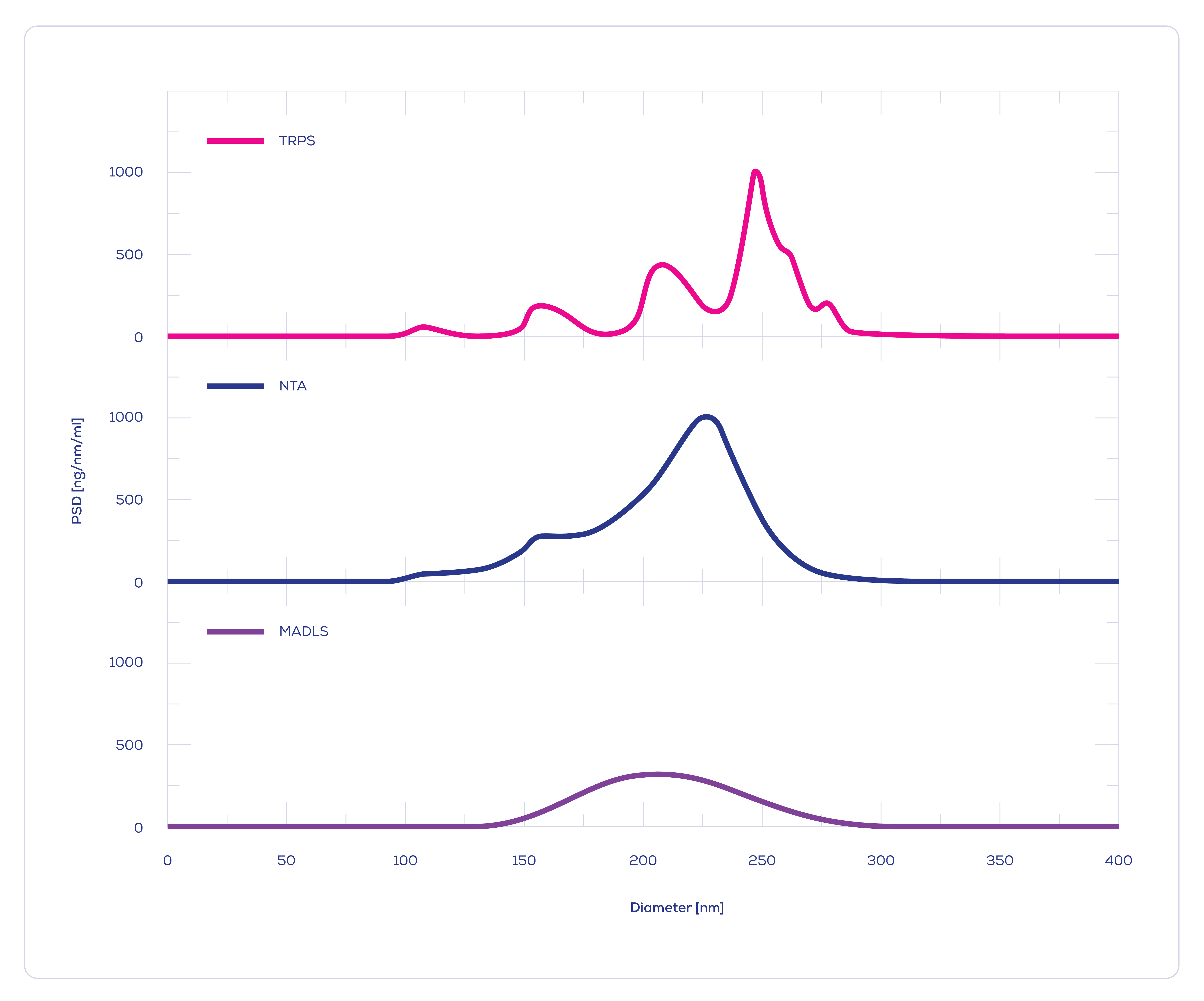
An opportunity for TRPS: success in the 220 nm-2 µm range
TRPS and CLS were the only methods that could provide accurate size and concentration data and correctly estimate the proportion of 220 nm and 2 µm subpopulations. As this size range is highly relevant for the analysis of NPs and MPs – and considering the time-consuming and laborious nature of CLS – this part of the study highlights the utility of TRPS for NP/MP-related studies. As discussed below, most techniques (DLS, TEM, FCM, SLS) struggled in this size range, while others were excluded for various reasons; NTA failed to resolve a 1 µm sample, therefore 2 µm would be well outside the detection limit. nFCM was excluded from the analysis of 220 nm and 2 µm particles as size and concentration detection limits are outside of the ranges of these samples. Meanwhile, 2 µm is beyond the upper detection limit of AF4-MALS.
DLS – the most widely used analytical technique for sizing measurements worldwide – underestimated the contribution of the 2 µm particles. This was surprising as DLS would usually be expected to overestimate larger particles. TEM was used to verify the size and confirm the spherical shape of particles in the bimodal samples; however it was not expected to provide an accurate assessment of concentration. Out of interest, Caputo et al. (2021) used TEM to count more than 3800 particles and found that instead of a 50:50 split, TEM estimated the 220 nm and 2 µm sample to be 12:88 w/w% – potentially as a result of smaller particles hiding behind larger particles. Meanwhile, SLS underestimated the size of the 220 nm population, and FCM could not distinguish either particle subpopulation.
Challenges, limitations, and a need to look beyond light scattering techniques
Ultimately, the analysis of NPs and MPs is highly challenging, and there is no single technique suited to covering the entire size range of all possible particle sizes. A number of techniques that have previously been recommended for the study of NPs and MPs (including DLS, FCM, NTA, AF4-MALS and nFCM) had suboptimal performances in this study. The authors note a particular need for techniques capable of covering the nanometre to micrometre interface, e.g. 800 nm to 10 µm.
As shown by the measurement of 220 nm and 2 µm particles, both TRPS and CLS are suited to covering a broad size range that is highly relevant for NP and MP analysis. Each of these two techniques have their limitations; for instance, two nanopore sizes were required to cover this range for TRPS, and CLS measurements require a precise knowledge of particle optical properties and sample density. Regardless, the authors recommend their use over other approaches. In the case of CLS, the required particle information will not always be available when analysing unknown environmental samples. As the authors suggest, the optimal choice will likely depend on the sample properties.
Importantly, the study also highlights the poor resolution of light scattering techniques that are commonly used and accepted by regulatory authorities, underscoring the need for high-resolution techniques more suited to implementing regulations around the use of primary NPs. As a technique capable of measuring particles at the lower nanometre range, and across a broad size range, TRPS is expected to become more widely used for MP and NP analyses.